Radiation is absorbed by humans in many ways and can harm the human body in the short and long term.
The way radiation enters the environment or human body can affect what health outcomes researchers expect to see. Nuclear toxicologists investigate the absorption, distribution, metabolism, and excretion (ADME) of radioactive materials in the environment to understand the exposure risks more fully and better predict resultant health outcomes. All these factors matter when it comes to understanding health harms.
Understanding absorption and target organs
There are many ways for people to be exposed to radiation—whether at their workplace, in their neighborhood, or by being near a nuclear facility whose safety measures have failed, causing a release of radioactive material.
We predominantly refer to radiation absorption pathways as either external or internal to the body. Both human absorption pathways have unique harms.
Internal exposures involve radioactive materials that directly enter the body during decay and can produce new radioactive decay products within the body. There are several types of internal exposure:
- Inhalation occurs when particles and radioisotopes enter the lungs through breathing and subsequently make their way into the bloodstream. This can happen during an accidental release of small airborne particles, as in the 1957 Rocky Flats fire, when plutonium was released from a Colorado processing facility.
- Dermal exposure involves direct skin contact with radioactive materials, or when radioactive particles enter an open wound. This becomes more likely in the workplace when appropriate personal protective equipment is not used.
- Submersion involves part or all of a limb, or an entire person, being submerged in radioisotopes. Lab workers use glove boxes to protect their health while assembling the plutonium pits that go into nuclear weapons, but when the gloves are torn or compromised, it can cause a limb to become partially or completely submerged in plutonium.
External exposures involve radiation near or around the body that is absorbed and can then affect normal bodily functions. External exposures do not create decay products in the body because the compound itself is not absorbed, just the radiation. External exposures can occur as a result of simply being near radioactive materials without any direct contact. The impacts of internal and external exposures will differ. For example, a uranium miner inhaling uranium that decays into other products within the lung (internal exposure) will experience different health effects than an atomic worker standing near radioactive waste and absorbing the radioactive particles being emitted (external exposure).
Though the air, clothing, and skin generally protect the body from certain types of radiation, they are not a defense against gamma rays emitted by radioactive materials. Gamma radiation is released in large quantities during nuclear weapon detonations or testing, but there are also naturally occurring gamma ray emitters such as americium-241, cesium-137, and cobalt-60, which are used in leveling gauges, smoke detectors, and medical equipment.
Radioactive materials interact with the body when absorbed
A basic concept of toxicology—the study of toxins in the environment—is absorption, distribution, metabolism, and excretion (ADME), which refers to the path that radioactive materials take within the human body and which tissues are most likely to be harmed. For example, the absorption pathway of inhalation is likely to target the lungs, but this is not guaranteed. Radiation can directly damage DNA and can also create new reactive chemicals once it has entered the body, which can alter normal bodily functions.
The distribution of radiation throughout the body, whether in a target organ or more systemically (e.g., circulating blood), can harm the human body through genetic alterations, tissue and organ damage, or by disrupting normal bodily functions. Radioisotopes can also be distributed and stored in multiple target organs.
There are many target organs for radioisotopes, including bones, teeth, fat cells, the thyroid gland, liver, kidneys, the reproductive system, and breast tissue . The decay of uranium-238 creates many radioactive products such as thorium-234 and -230, radium-226, radon-222, and polonium-218 and -214. Each of these products target different organs: thorium-230 is predominantly stored in bone, but polonium is distributed in soft tissue and bone, and radium is stored on the surface and the marrow of bones. All are decay products of each other that target different areas of the body.
Biological markers (or biomarkers) can be used to understand how toxicants are distributed in the body and in target organs such as the baby teeth used in the St. Louis Baby Tooth Study.
This study found children born in the mid-1960s had levels of strontium contamination 60 times higher than those born in the early 1950s (a 100-fold increase). This work was conducted across the greater St. Louis area by the Committee for Nuclear Information and Washington University faculty. Researchers measured strontium-90 in more than 320,000 baby teeth over a dozen years. Strontium-90 is more easily measured in children due to their increased radiosensitivity and can therefore be measured in bones and teeth (which strontium-90 targets) without having to do something invasive like taking blood or a bone biopsy.
The high levels of strontium-90 in this population have been attributed to radioactive fallout from aboveground nuclear testing. This region has also experienced increased incidence of low birth weights and childhood cancers among people living there.
Metabolism refers to any of the body’s many processes that attempt to recognize unfamiliar hazards such as chemicals or radiation. It is important to understand these biological defenses are not as relevant in high doses of radiation exposure, which are likely to cause irreparable damage or death.
Many of the body’s metabolic processes that maintain normal functioning often depend on specific proteins (hard-coded in our genetic material, including DNA and RNA) called enzymes. When radiation directly harms our genetic material, these enzymes can be altered and therefore normal bodily functions are altered as well.
It is important to understand that some radioactive materials, such as uranium, cause both radioactive harms (such as tissue scarring and alterations to genetic material that can induce cancers) and chemical harms. As a heavy metal, uranium is part of a group of chemicals known to harm neurological function, child development, and birthing outcomes.
Excretion refers to how these harmful materials are removed either from circulation (stored in target organs or removed with treatment) or removed from the body due to natural processes (such as urine, feces, vomit, and more). Excreted exposure levels can be measured to understand internal contamination (again, these are called biomarkers). Examples include tritium excreted through the urine, or the severe vomiting symptoms characteristic of radiation poisoning.
Exposure duration affects health outcomes
The frequency and duration of exposure to ionizing radiation play key roles in what health outcomes may result. We often define these exposures as acute or chronic.
Acute exposure typically occurs at one point in time, with moderate to high contamination levels. For example, in the Hiroshima and Nagasaki atomic bombings, many hibakusha—or bomb-affected people—were left living with radiation sickness, cancers, autoimmune disease, and other chronic illnesses from experiencing a large, sudden dose of radiation in their environment.
Due to the long half-lives of some fissile materials, it is important to understand that while the majority of radiation from aboveground testing, accidental detonations, and bombings dissipates shortly after detonation, radiation from nuclear waste leakages and other long-term releases can persist in the environment for many years.
Chronic exposure to radiation is defined as occurring or repeating over a long period of time. A low dose is often assumed because high doses are likely to result in death and are not epidemiologically trackable over longer time periods. Many frontline communities—those near nuclear testing or mining and production sites—have spoken out about the health harms they have experienced from chronic low-dose exposure, and radiation health researchers are still trying to catch up.
More science is needed on low-dose radiation
The National Academies of Science, Engineering, and Mathematics (NASEM) recently reported on low-dose radiation (<100 mGy or <5 mGy/hour) and human health, stating it is more difficult to understand the effects of low concentration levels of radiation because of the long time between exposure and cancer development.
A 2022 report from the National Academies of Science (NAS) suggested creating tools for more sensitive detection of radiation and precise characterization of cell and tissue changes, but there are logistical and financial hurdles to this approach, such as figuring out how to harmonize new low-dose radiation data across national health research databases.
NASEM reported being excited about these additional proposals :
- More research into non-cancer health outcomes
- Additional focus on differential impacts (i.e., how exposure might differ based on gender, age, lifestyle, ethnicity, etc.)
- The importance of involving frontline communities in research, including appropriate collaboration with Indigenous nations
Recent research has associated low-dose radiation with increased cancer deaths. Specifically, with a lag time of 10 years for cancer development, mortality due to solid cancers increased with cumulative dose over time by 52 percent per Gy.
Related explainers
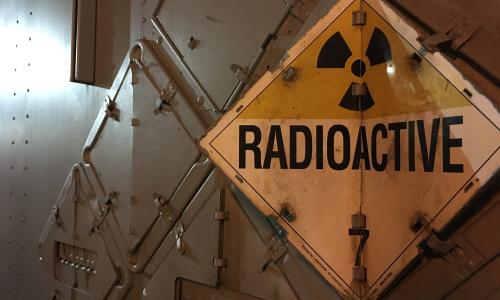
Understanding Radiation
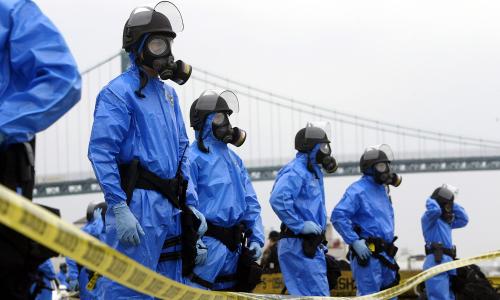
Where Radiation Comes From
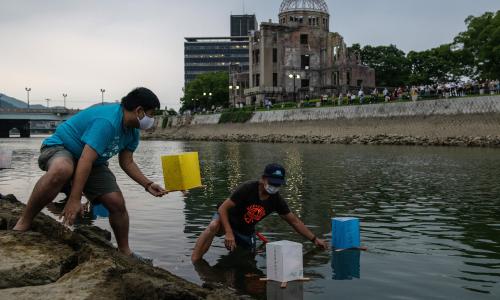
Health Impacts of Radiation Exposure
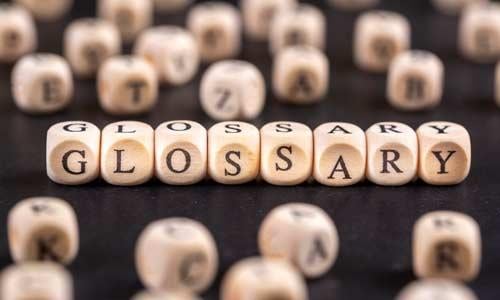
The Language of Radiation: A Glossary
The Union of Concerned Scientists has worked on nuclear weapons-related issues for more than 50 years. Get involved here.